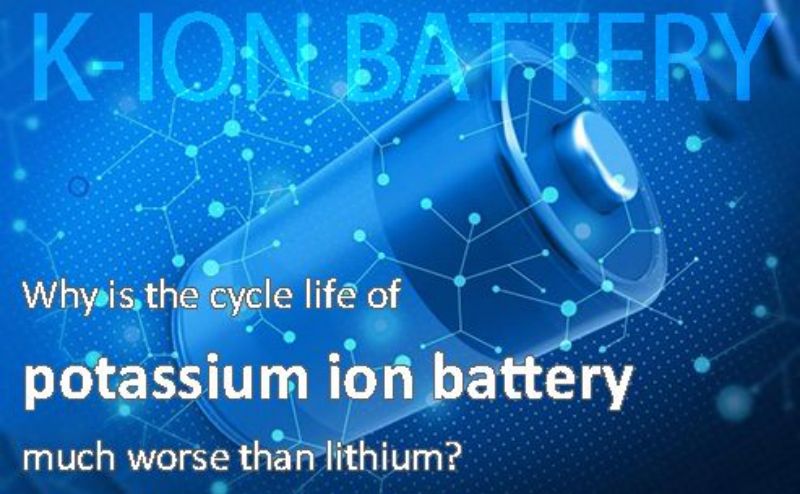
Main content:
The commercialization of lithium-ion batteries (LIBs) was successfully facilitated in the late twentieth century by graphite replacing lithium metal anodes through the formation of LiC6 (bibinary stage lithium graphite intercalated compound (GIC)). In 2015, a breakthrough was reported in natural graphite by forming a similar binary GIC (KC8) as an anode material for potassium ion battery (PIBs). As is often discussed today that lithium vs sodium battery who isbetter, because of their lower cost and considerable energy density, potassium ion battery is considered the most competitive candidates for large-scale stationary energy storage.
Conventional carbonate electrolytes consisting of vinyl carbonate (EC) and linear carbonate are most widely used in potassium ion battery and lithium ion battery. Solid electrolyte interphase (SEI) formed on graphite surface can prevent irreversible side reactions caused by reducing decomposition of electrolyte. This EC-based SEI is mainly composed of organic components and small amounts of inorganic substances, such as fluoride, metal oxides, which are mainly derived from the decomposition of salts.
The cycle stability of graphite in potassium ion battery is worse
However, there is an obvious problem in that the graphite anode in potassium ion battery shows worse battery cycle stability than in lithium ion. As far as this problem is concerned, two possible reasons have now been suggested. One is that the volume expansion of graphite after K+ ion intercalation is large (about 60%), which will lead to the distortion of graphite microcrystal structure. Another is the relative instability of the SEI on the graphite surface. However, these two causes have not been thoroughly identified.
Around 2010, a concentrated electrolyte strategy was proposed, which was subsequently applied to potassium ion battery or lithium ion battery. SEI, derived from a stable anion on the graphite anode, is formed after the first cycle in highly concentrated electrolytes based on various solvents (i.e., ether, acetonitrile, linear carbonate or phosphate esters, etc.). Thus, the dissolved Li+ or K+ ions must be desolvated before intercalation and form a binary GIC, that is, LiC6 or KC8. More interestingly, the graphite anode exhibits outstanding cyclic stability in both lithium ion and potassium ion battery. Obviously, this is in sharp contrast to the cyclic properties of graphite in ester based electrolytes. For potassium ion battery, the graphite anode showed worse cycling performance in the diluted ester based electrolyte than in the high concentration electrolyte, although both formed binary GICs. Unfortunately, this intriguing phenomenon has not attracted people's attention until now.
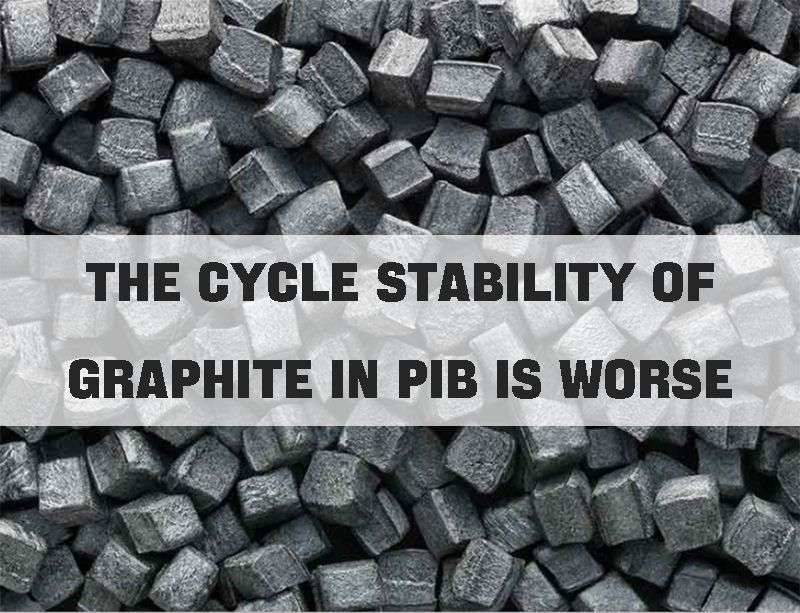
Studies have found the key factors affecting the cyclic stability of graphite in potassium ion batteries
Recently, the graduate school of tsinghua university in shenzhen international kang unisoft professor, associate professor of ZhaiDengYun et al., reveals the "decision of potassium ion battery graphite anode cycle stability of the key factors" their research results show that in the PIBs, larger volume expansion is not the leading cause of serious attenuation capacity of graphite anode, and the stability of the SEI is the key to maintain a high capacity. When EC/DEC electrolyte was applied to PIB, a cumulative SEI containing an oligomer component was produced on the graphite surface.
Continuous SEI accumulation hinders the intercalation and delamination of K+ ions, which eventually leads to the capacity decay of the graphite anode. This work provides a clearer explanation of the graphite capacity loss in the potassium ion battery and a deeper understanding of the critical influence of a stable SEI on the cyclic stability of the graphite anode. The work was published in the international top magazine ACS Nano under the title Key Factor Determining the Cyclic Stability of the Graphite Anode in Gigao-ion Batteries.
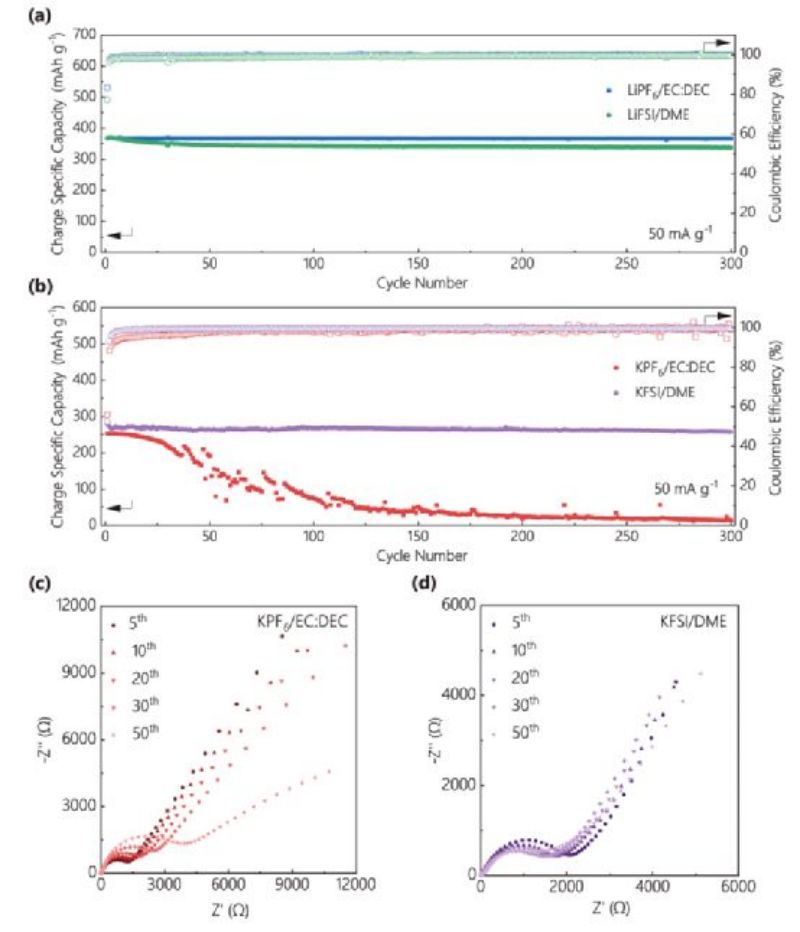
FIG. 1. Cycling stability of (a)Li/Graphite half cells and (b)K/Graphite half cells in different electrolytes. EIS curves of K/Graphite half cells after different cycles in (C)KPF6/EC:DEC and (D)KFSI/DME electrolytes.
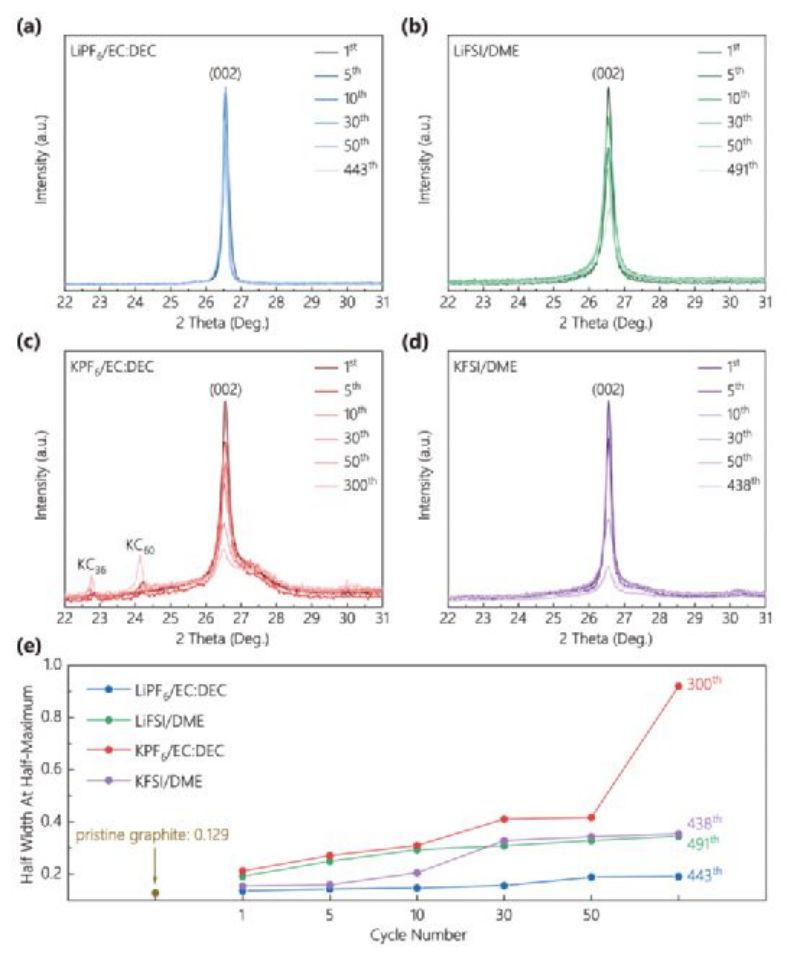
Figure 2. XRD patterns of graphite after different cycles in (A) LiPF6/EC:DEC, (b) LiFSI/DME, (c) KPF6/EC:DEC, and (d) KFSI/DME electrolytes. (e) Half-width and half-height values of (002) peak after different cycles in the four electrolytes.
The graphite anode in potassium ion battery maintains almost the same high crystallinity as that in lithium ion battery. Thus, an obvious conclusion is that the greater volume expansion of graphite induced by intercalated K+ ions does not lead to severe distortion of the highly ordered graphite structure when stable SEI forms on the graphite surface, unlike previous understanding. The graphite anode undergoes the same volume expansion but exhibits significantly different cyclic properties. Compared with the volume expansion of graphite anode during intercalation and delamination, the SEI stability of graphite surface may have a more important influence on the cyclic stability of graphite.
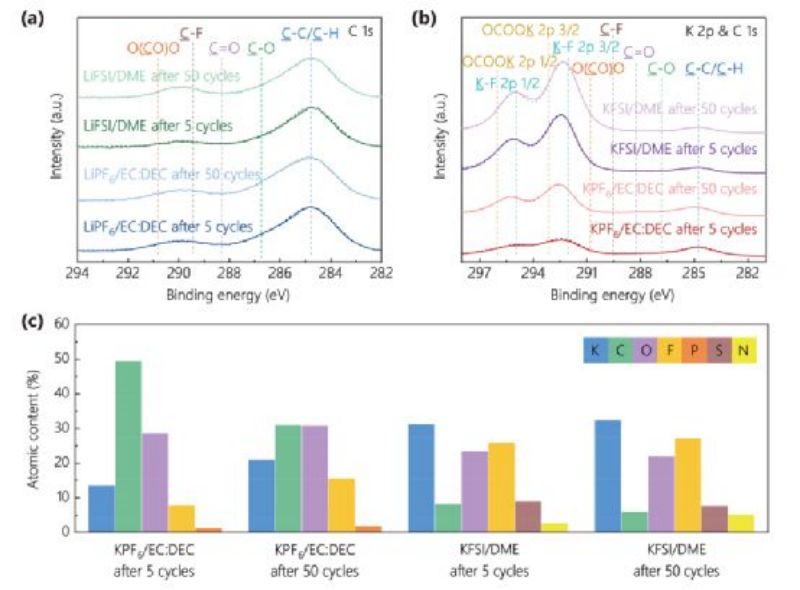
Figure 3. Deconvolved K 2p and C 1s XPS spectra of SEI on graphite surfaces after different cycles in (a)LiPF6/EC:DEC and LiFSI/DME electrolytes and (b)KPF6/EC:DEC and KFSI/DME electrolytes. C) Atomic proportion of SEI on graphite anode after cycling in potassium electrolyte.
In the KPF6/EC:DEC electrolyte, the atomic content of the graphite surface after 50 cycles is highly variable compared with that after 5 cycles, whereas the atomic content of the other three electrolytes is significantly less variable. The instability of SEI on graphite surface in KPF6/EC:DEC electrolyte was confirmed. Comparing the C 1s/K 2p and C 1s spectra of graphite surface in four different electrolytes, it can be found that after 50 cycles in KPF6/EC:DEC electrolyte, the intensity of the peak corresponding to SEI components (K-F, OCOOK, etc.) increases significantly, indicating that SEI on graphite surface may accumulate continuously during cycling. The accumulation of SEI resulted in a gradual increase in the charge transfer impedance at the anode/electrolyte interface during cycling (FIG. 1c).
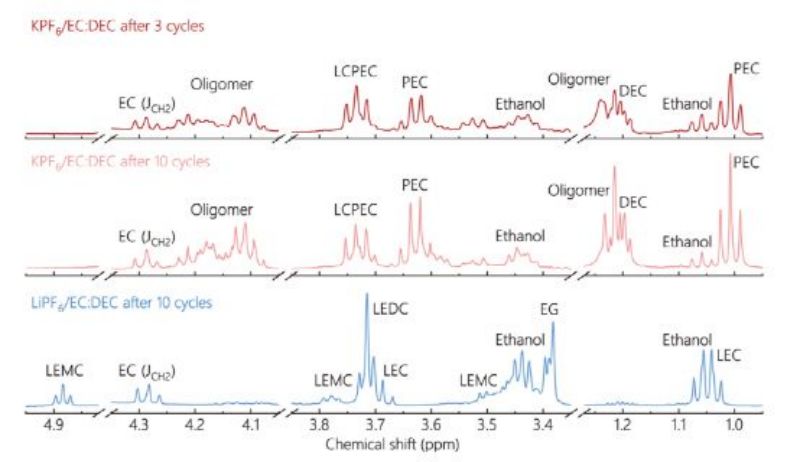
Figure 4. NMR spectra of SEI in KPF6/EC:DEC electrolyte after 3 cycles and 10 cycles, and of SEI in LiPF6/EC:DEC electrolyte after 10 cycles.
XPS and NMR composition analysis showed that the relative content of oligomers on the surface of potassium electrographite increased greatly after cycling. Continuous accumulation of oligomer components in SEI will lead to a decrease in ionic conductivity of SEI and block ion diffusion through SEI. Therefore, it hinders intercalation and delamination of K+ ions, leading to the residue of K-Gics at higher stages and the capacity decay of the graphite anode. In contrast, lithium ethylenebicarbonate (LEDC), lithium ethylenebicarbonate (LEMC) and lithium ethylenebicarbonate (LEC) were found in SEI formed in LiPF6/EC:DEC (FIG. 4). Therefore, whether SEI is deposited in EC:DEC electrolytes containing oligomer components may be the main difference in SEI between potassium ion battery and lithium ion battery, and further become a key factor in determining SEI stability.
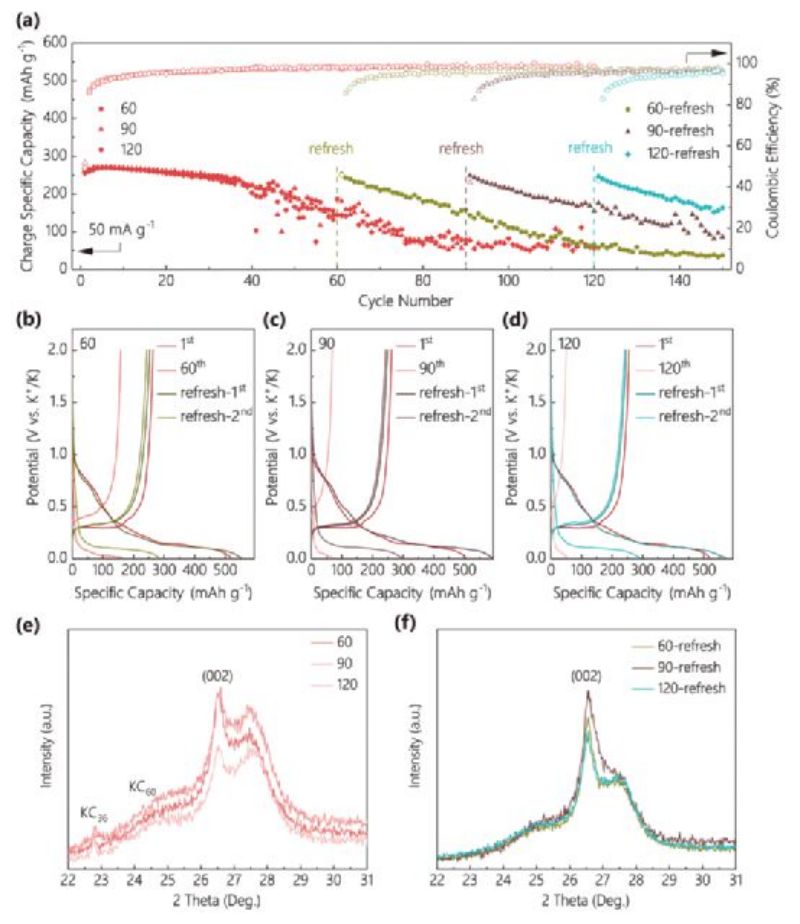
Conclusion
- Firstly, the cycle stability of graphite anodes using traditional dilute ester or high concentration ether electrolytes in lithium ion battery or potassium ion battery was thoroughly compared, and the fundamental reason for the serious battery capacity decay of graphite anodes using KPF6/EC:DEC electrolyte in PIB was emphatically discussed.
- The results show that the key factor in determining the cyclic stability of graphite anode should be the stability of SEI formed on the graphite surface, rather than the large volume expansion of graphite caused by intercalation and delamination of K+ ions.
- SEI on the graphite surface for potassium ion battery accumulates during cycling in KPF6/EC:DEC electrolyte, in contrast to the stability of SEI in LiPF6/EC:DEC electrolyte for LIB and in high concentration DME electrolyte. At the same time, it was found that the continuous formation of an oligomer was the main reason for SEI accumulation in KPF6/EC:DEC electrolyte. A large amount of SEI prevents K+ ions in potassium ion battery from entering or unplugging from the graphite anode, resulting in a rapid decline in the capacity of the graphite anode.
- This work elucidates the key factors that determine the cyclic stability of graphite anodes and provides a clearer direction for improving the capacity retention of graphite anodes with LIB or PIB.
Paper source:
Key Factor Determining the Cyclic Stability of the Graphite Anode in Potassium-Ion Batteries
ACS Nano ( IF 18.027 ) Pub Date : 2022-08-09 , DOI: 10.1021/acsnano.2c03955
Fu Yuan, Junyang Hu, Yu Lei, Rongyi Zhao, Chongwei Gao, Huwei Wang, Baohua Li, Feiyu Kang, Dengyun Zhai