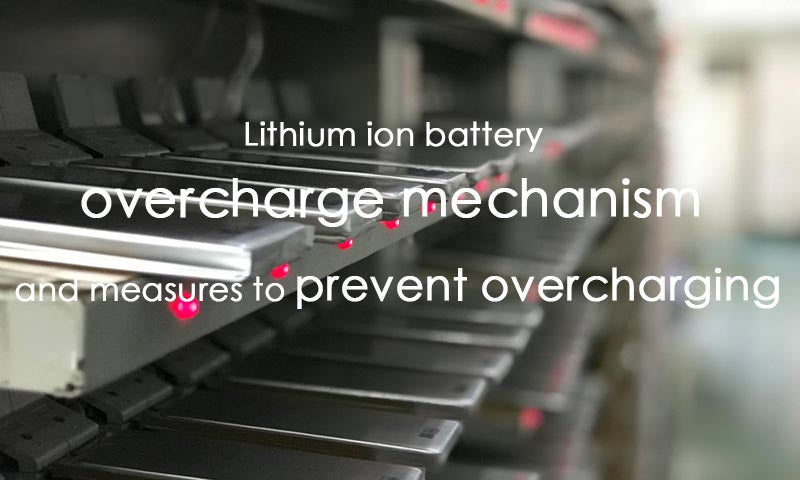
Main content: |
Overcharge is one of the reasons that the performance of lithium ion battery is affected in the process of using it. Therefore, it is necessary for us to understand the underlying mechanism of lithium ion battery overcharge and the current measures to prevent overcharge.
1.Lithium ion battery overcharge process and mechanism
In this paper, the overcharge performance of a NCM111+LMO 40Ah pouch battery was studied by experiment and simulation. The overcharge current was 0.33C, 0.5C and 1C, respectively. The battery size is 240mm * 150mm * 14mm. (Calculated according to the rated voltage 3.65V, the volume specific energy is about 290Wh/L, which is relatively low)
The changes of voltage, temperature and internal resistance in overcharging process are shown in FIG. 1. It can be roughly divided into four stages:Heat generation in the process of lithium ion battery overcharge includes reversible entropy heat, joule heat, chemical reaction heat and heat released by internal short circuit. The chemical reaction heat includes the heat released by Mn dissolution, reaction between lithium metal and electrolyte, electrolytic liquid oxygenation, SEI film decomposition, negative electrode decomposition and positive electrode (NCM111 and LMO) decomposition. Table below shows the enthalpy change and activation energy of each reaction. (Side effects of binder are ignored in this paper)
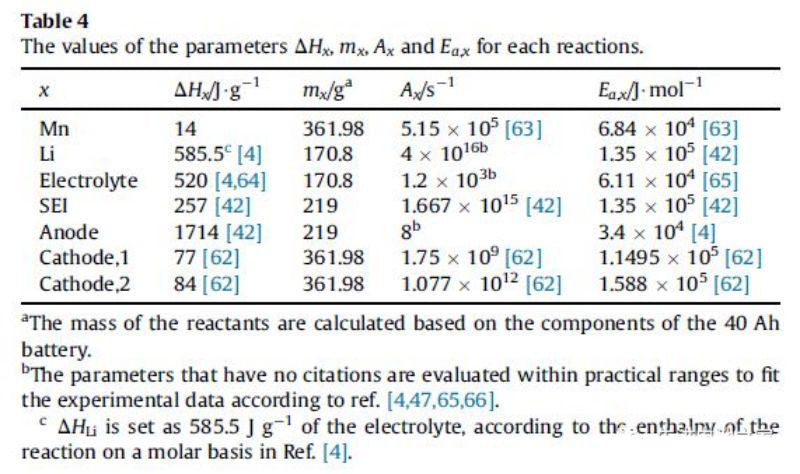
FIG. 3 is the comparison of heat production rate under overcharging of different charging currents. The following conclusions can be drawn from FIG. 3:
1) With the increase of charging current, the thermal runaway time is advanced.
2) The heat generation in the process of lithium ion battery overcharge is mainly joule heat. SOC<1.2, the total heat produced is basically equal to joule heat.
3) In the second stage (1<SOC<1.2), Mn dissolution, lithium metal reaction with electrolyte, electrolytic liquid oxygenation three kinds of side reactions began to react successively. At 1C, the reaction moves ahead.
4) When SOC>1.45, the heat released by lithium metal reacting with electrolyte will exceed joule heat.
5) When SOC>1.6, the decomposition reaction of SEI film and anode begins, the heat production rate of electrolytic liquid oxygenation reaction increases sharply, and the total heat production rate reaches the peak. (The descriptions of 4 and 5 in the literature are somewhat inconsistent with the figure, so adjustments are made here based on the figure.)
6) In the overcharging process, the reaction of lithium metal with electrolyte and electrolytic liquid oxygenation are the main reactions.
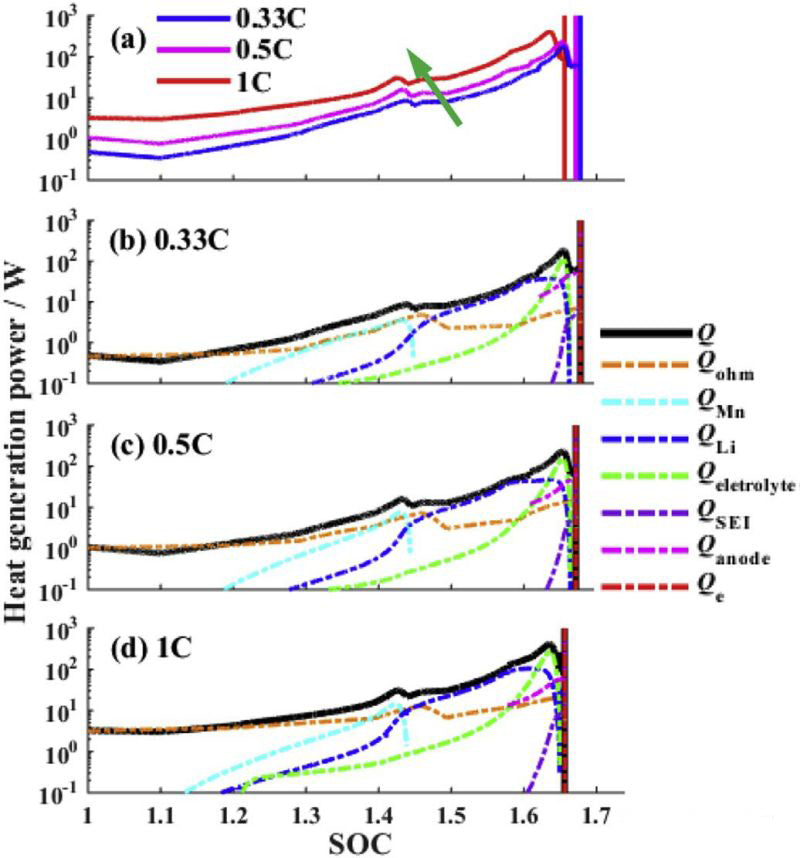
According to the above analysis, the electrolytic liquid oxygenation potential, the negative electrode capacity and the initial temperature of thermal runaway are three key parameters of overcharge. FIG. 4 shows the influence of three key parameters on lithium ion battery overcharge performance. It can be seen that the increase of electrolytic liquid oxygenation potential can greatly improve the overcharge performance of the battery, but the negative electrode capacity has little effect on the overcharge performance. (In other words, the high voltage electrolyte can improve the battery overcharge performance, and the increase of N/P ratio has little effect on the battery overcharge performance.)
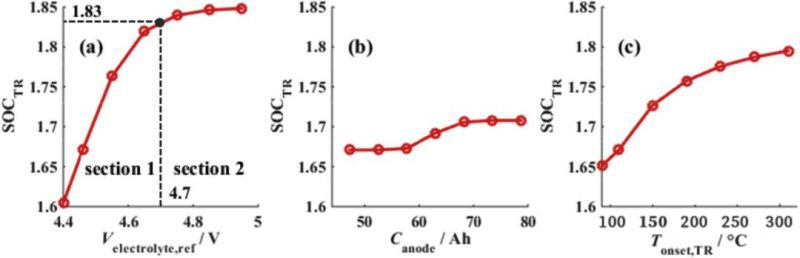
Reference: D. Ren et al. Journal of Power Sources 364(2017) 328-340
2.Changes in voltage and temperature of LCO batteries during overcharge
In this part we introduce the changes of voltage and temperature of lithium cobalt acid battery when it is overcharged. The figure below is the overcharge voltage and temperature curve of lithium cobalate battery, and the horizontal axis is the amount of delithium. The anode is graphite and the electrolyte is EC/DMC. The battery capacity is 1.5Ah. The charging current is 1.5A and the temperature is the internal temperature of the battery.
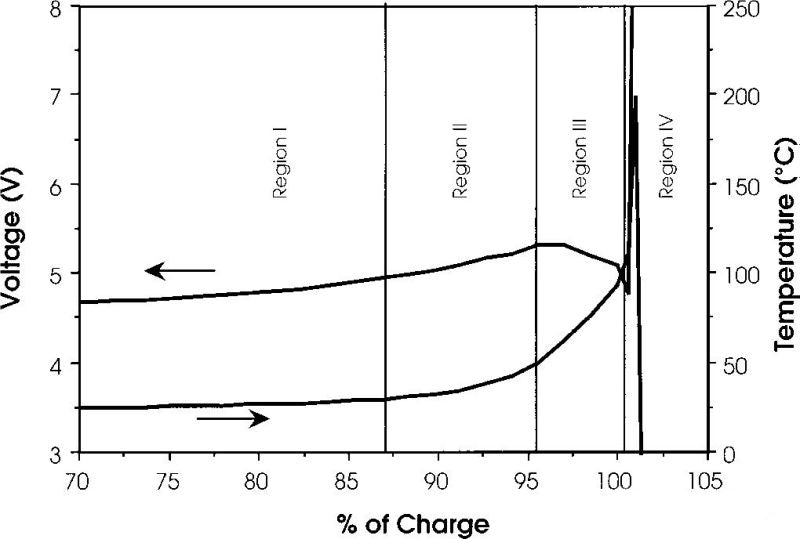
Lithium ion battery overcharge can be roughly divided into four region, and the characteristics of each area are as follows:
Region I
1. The battery voltage rises slowly. More than 60% of lithium cobalt oxide was removed from the positive electrode, and lithium metal was precipitated on the negative electrode side.
2. Battery bulge may be due to electrolyte oxidation at high pressure on the positive side.
3. The temperature is basically stable with a slight increase.
Region II
1. The temperature begins to rise slowly.
2. In the range of 80-95%, the positive electrode impedance increases, and the internal resistance of the battery increases, but it decreases in 95%.
3. The battery voltage exceeds 5V and reaches the maximum.
Region III
1. At about 95%, the battery temperature begins to rise rapidly.
2. The battery voltage drops slightly from about 95% until it approaches 100%.
3. When the internal temperature of the battery reaches about 100 ° C, the battery voltage drops sharply, which may be caused by the decrease of the internal resistance caused by the rising temperature.
Region IV
1. When the internal temperature of the battery is higher than 135 ° C, the PE diaphragm begins to melt. The internal resistance of the battery increases rapidly, the voltage reaches the upper limit (~12V), and the current drops to a lower value.
2. Between 10-12v, the battery voltage is unstable and the current also fluctuates.
3. The internal temperature of the battery rises rapidly and reaches 190-220℃ before the battery is broken.
4. The battery is broken.
Ternary battery overcharge is similar to lithium cobalate battery. When ternary square aluminum shell battery is overcharged on the market, OSD or CID is roughly controlled to start when entering zone III to cut off current and protect the battery from overcharge.
Reference:Journal of The Electrochemical Society, 148 (8) A838-A844 (2001)
3.Measures to prevent lithium ion battery overcharging
FIG. 6 shows voltage and temperature curves of NCM+LMO/Gr system battery under overcharge. The maximum voltage is reached at 5.4V, followed by a voltage drop that eventually leads to thermal runaway. The voltage and temperature curves of lithium ion battery overcharge are very similar.
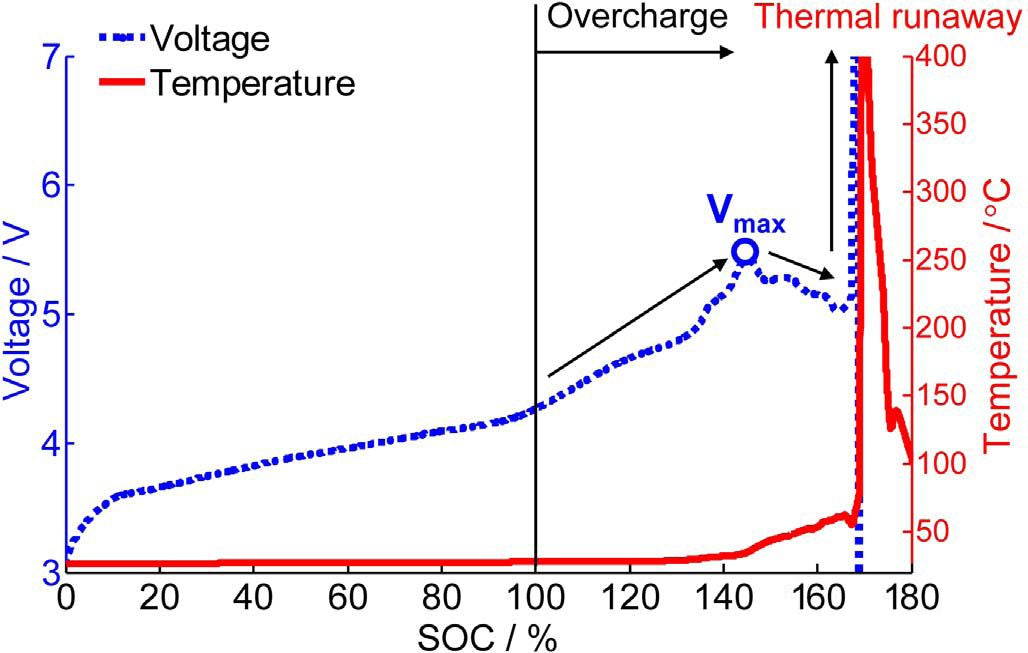
When the condition of lithium ion battery overcharge is happening, heat and gas are generated, including ohmic heat and heat generated by side reactions, of which ohmic heat is the main. The side reaction of the battery caused by overcharge is that excessive lithium is embedded into the negative electrode, and lithium dendrites will grow on the surface of the negative electrode (N/P ratio will affect the initial SOC of lithium dendrite growth). Second, excess lithium escapes from the positive terminal, causing the structure of the positive terminal to collapse, releasing heat and oxygen. Oxygen will accelerate the decomposition of the electrolyte, the battery internal pressure continues to rise, after a certain degree of safety valve open. Contact between the active substance and the air will generate further heat.
Studies have shown that reducing the amount of electrolyte can significantly reduce the heat and gas production during overcharge. In addition, it has been studied that the battery without splint or safety valve can not be opened normally when overcharged, and the battery is prone to explosion.
Slight overcharging does not result in thermal runaway, but in capacity decay. It is found that when the BATTERY with NCM/LMO hybrid material as positive electrode is overcharged, the SOC is lower than 120% and the SOC is higher than 130%, but the SOC has no obvious attenuation.
At present, there are several methods to solve the overcharging problem:
1) Set the protection voltage in BMS, which is usually lower than the peak voltage during overcharge;
2) Improve the anti-overcharge capability of the battery through material modification (such as material coating);
3) Add anti-overcharge additives, such as REDOX pair, to the electrolyte;
4) With the use of voltage-sensitive film, the film resistance is significantly reduced when the battery is overcharged, which plays a shunt role;
5) OSD and CID designs are used in square aluminum shell batteries, which are currently universal anti-overcharge designs. A similar design cannot be achieved with soft-pack batteries.
Reference:Energy Storage Materials 10 (2018) 246 -- 267