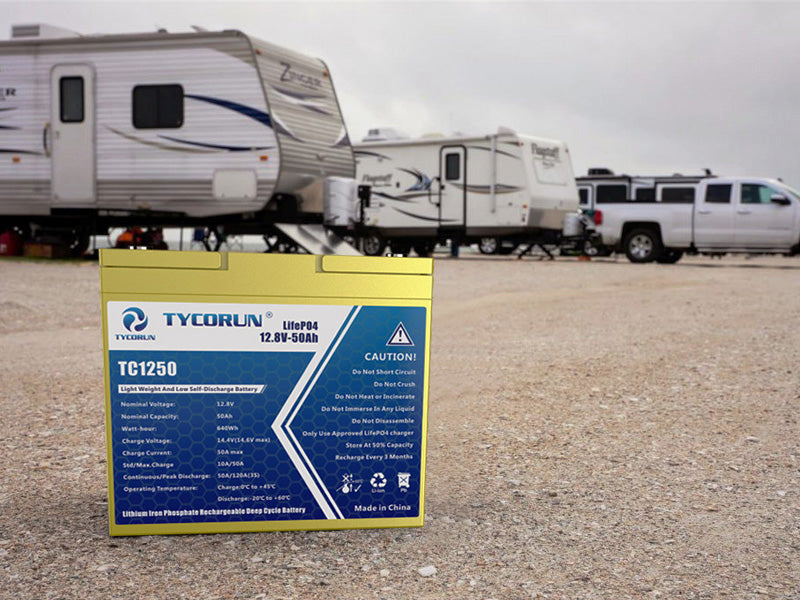
Main content:
At present, the research on anode materials for lithium-ion batteries mainly focuses on the nano-scale synthesis of compounds (to shorten the diffusion distance of Li+ ions), and at the same time, the ratio of particle size to specific surface area on the anode materials falls within a suitable range to ensure that as much as possible High electrode density [due to particle size reduction, resulting in a corresponding increase in interfaces (including between current collector and active material, and between active material and active material) and reduced electrode density (high specific surface area); while This just offsets the effect of increased diffusion distance and decreased conductivity due to particle size reduction].
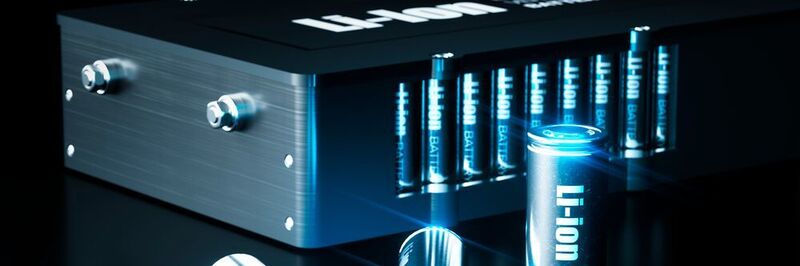
1. Short-term development of anode materials
The characteristics of Li4Ti5O12 make it attractive as a cathode active material for power lithium batteries, especially the redox reaction potential of Ti4+/Ti3+ is relatively high, about 1.55V/Li+/Li, which is greater than that of Li+/Li. This high potential will make the electrolyte less prone to decomposition, smaller volume change during reduction and oxidation (lithium deintercalation process), and better electrochemical reversibility, thereby achieving excellent charge-discharge rates.
During the initial lithium intercalation and subsequent charge-discharge cycles, the expansion or contraction of the grid is minimal. In fact, the volumes of the oxidized phase (i.e. the delithiated phase LLi4Ti5O12 ) and the reduced phase (i.e. the lithiated phase Li7Ti5O12 ) are almost identical (ΔV = ±0.07%). It is for this reason that Li4Ti5O12 is considered a "zero strain" material. Therefore, good adhesion between the inside of the electrode, between the electrodes, and between the separator and the current collector can be maintained during the entire charge-discharge cycle. This allows the battery to avoid cracks and damage that can lead to reduced capacity and performance. The theoretical capacity density of this type of battery can reach 175mA·h/g.
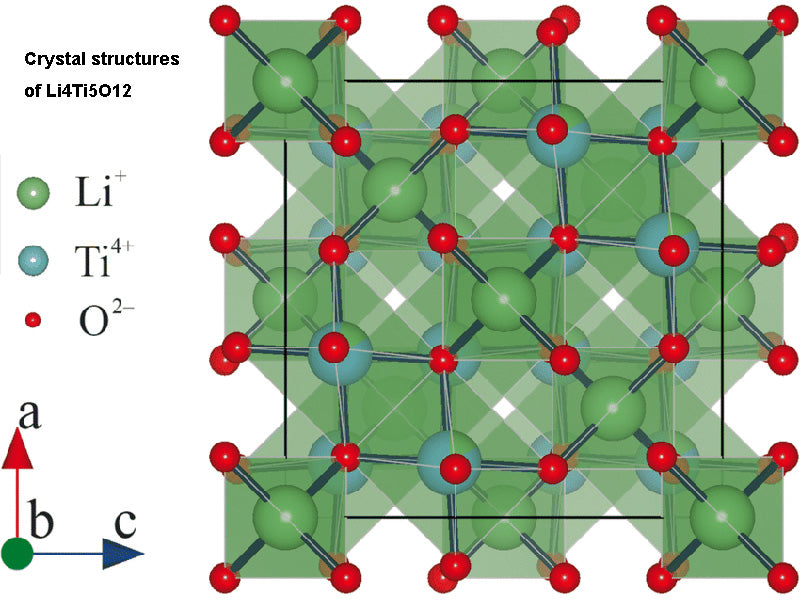
In fact, when the battery is operated at low rate, its recoverable capacity after cycling is close to the above value (ie, 175 mA h/g). By controlling the preparation method and particle morphology of the electrode material, better rate performance can be obtained. The potential in the process of lithium intercalation is higher than the decomposition potential of the electrolyte, which means that an inert layer cannot be formed between the electrode and the electrolyte interface, thereby causing the electrolyte to decompose. Moreover, this negative electrode material has strong thermal and chemical stability, can be charged and discharged at a high rate, and/or can work at a lower ambient temperature.
In addition, the intercalation potential of these anode materials is high compared to the lithium deposition potential, so there is no risk of internal short circuits under high current charging conditions. Working at this potential, aluminum material can be used as the current collector of the negative electrode material, which will be lighter than copper material (copper material is often used as the current collector of graphite electrodes). However, as a negative electrode material, its relatively high potential prevents the battery from obtaining the high energy density when using graphite electrodes. Currently, Li4Ti5O12 is a commercialized zero volume expansion anode materials for the preparation of lithium-ion batteries with high power characteristics and fast charging capability. Batteries with these anodes materials are being promoted for use in portable tools, smart cards or electrical drive systems.
However, the actual achievable capacity of Li4Ti5O12 lithium batteries is close to its theoretical value (175 mA h/g), but it is still lower than that of lithium batteries using graphite as the anode materials (330 mA h/g), and the energy There is limited room for improvement in density. Similarly, some TiO2 with special structures can also be used as negative electrode materials (especially B or H). The compactness of these TiO2 structures is weaker than that of anatase TiO2; compared with the currently used graphite electrodes, the use of such negative electrode materials can Similar advantages to using Li4Ti5O12 are obtained.
However, the theoretical capacity of this type of anode materials are significantly higher than that of lithium titanium oxide [TiO2(B) is 338 mA h/g, while Li4Ti5O12 is only 175 mA h/g]. Thus, for electrodes with low surface capacity (<0.5 mA h/cm2), the maximum achievable capacity is 260 mA h/g when operating at a very small charge-discharge rate (C/100). Recent studies have achieved 60% of the above-mentioned capacity values in practical low-rate charge-discharge (C/10), and the latest study by several authors achieved 75% of the theoretical capacity at high rate discharge at 10C using TiO2 nanowire technology . Particle size, morphology, specific surface area and microstructure will be key factors for performance improvement.
2. Mid-term development of anode materials
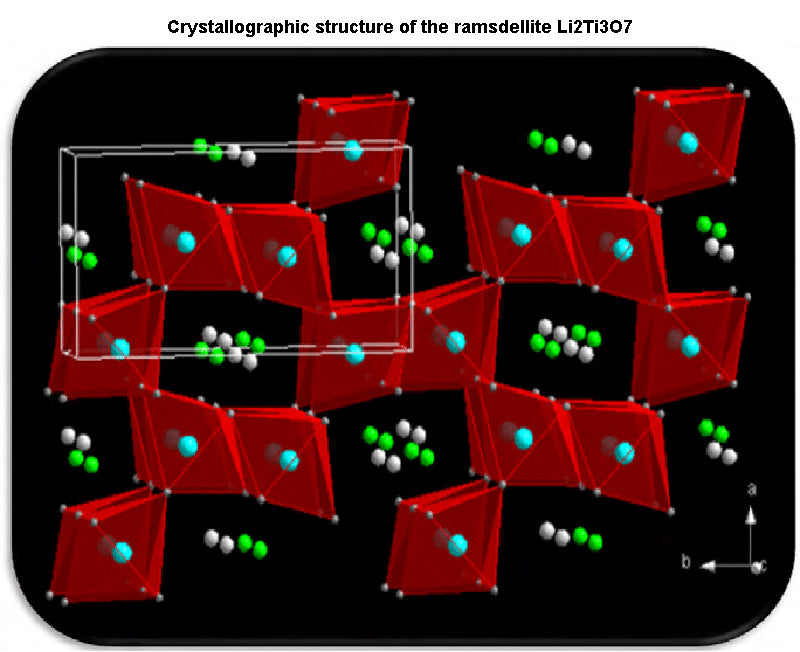
Other anode materials have also received high attention in the past few years, including a variety of possible titanium oxides (Li4Ti5O12、Li2Ti3O7、TiO2, etc.), which can form different nanostructures according to different synthesis conditions: nanoparticles (50 ~100nm), nanowires, etc. Based on their (Li4Ti5O12 or TiO2(B)) morphological features (nanomaterials, large specific surface area, etc.), these anode materials can achieve high charge-discharge capacity, which makes them a non-stop between batteries and supercapacitors Optimal anode materials for symmetric energy storage devices.
Moreover, by manipulating the morphology and nanostructure of these anode materials using conventional synthesis methods, it is possible to improve the anode materials properties and obtain capacities close to those of graphite electrodes. In addition, optimizing the activation environment inside the electrode composites will help strengthen the intrinsic properties of these compounds and enable thicker anode materials electrode to be coated (which will allow them to have a larger surface capacity, 1 mA h/cm2) , thus, can bring high energy density and power density.
3. Long-term development of anode materials
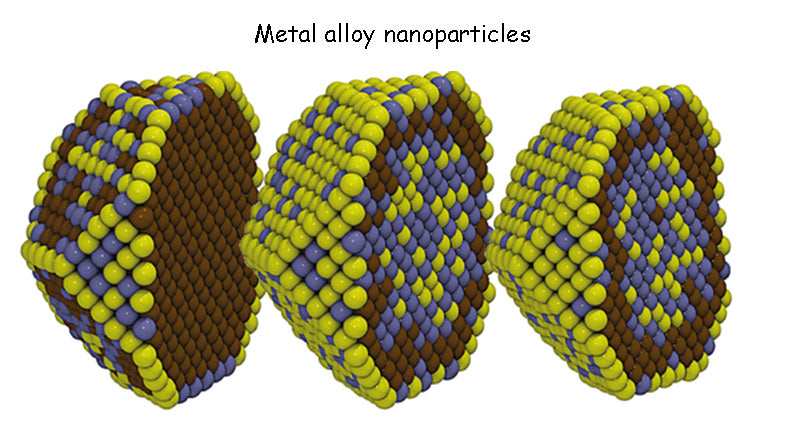
There is extensive research into finding alternatives to graphite anode materials currently in use, including silicon, tin and metal alloy nanoparticles. It is foreseeable that the specific capacity of 350 mA h/g of graphite anode materials can be exceeded, and can reach a value of more than 1000 mA h/g (theoretically it can reach 3800 mA h/g). Different materials are synthesized with nano-silicon in the form of thin films or particles, and added to the conductive carbon matrix by deposition or embedding, etc., which are expected to obtain the above-mentioned good properties.
However, the optimization of electrodes, and the overall optimization of batteries containing these compounds, still requires years of research. The volume expansion of Si-Li alloy, the isolation (passivation) of the contact between particles, etc. are still problems to be solved. Finally, the potential of these anode materials is at 0.5V/Li+/Li, which is very close to that of graphite. However, although these anode materials can achieve high energy densities, their risks during fast charging limit the available capacity of batteries (increased lithium dendrites can lead to serious safety concerns).
Read more: Development of cathode materials for lithium- ion batteries