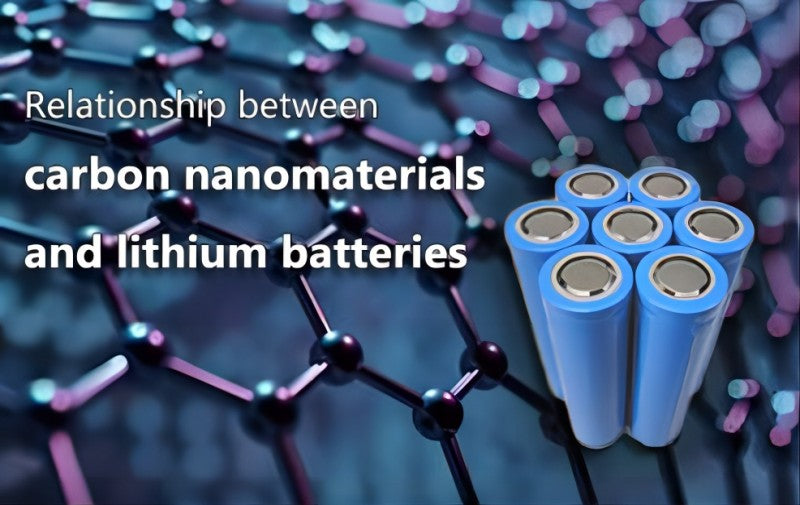
Main content:
There are many new blood in the carbon material family, such as carbon black, graphene, fullerene, carbon nanotubes, graphyne, carbon nanodots, porous carbon, carbon nanofibers. Nano-carbon materials have extremely high carrier mobility, excellent electrical conductivity, high thermal conductivity, super strong mechanical properties and unique light transmittance. Smart home and biomedical fields have important application prospects.
The electrochemical performance of lithium-ion batteries largely depends on the composition, microstructure, and morphology of electrode materials. From petroleum coke, to the emergence of graphite electrodes, and to the popularization of commercial graphite, carbon materials play an important role in the composition of lithium-ion batteries. With the continuous improvement of people's performance requirements for lithium-ion batteries, traditional carbon materials are gradually difficult to meet the needs of practical applications.
After the emergence of new nano-carbon materials, it was found that these nano-carbon materials can have important applications in lithium-ion batteries. For example, a high specific surface area can provide more lithium storage sites for lithium ions, shorten the transmission path of lithium ions to improve the diffusion and deintercalation rate of lithium ions, and increase the electron transfer rate of carbon materials. The diversified performance of nano-carbon materials makes it a hot spot in the application research of lithium-ion batteries.
Application of carbon nanomaterials
Construction of conductive network
Constructing an excellent electron transport network is crucial for lithium-ion batteries. Nano-carbon materials have high electron mobility and are good conductors of electrons. How to construct a perfect electron transport network inside the electrode and between the electrode and the external circuit is one of the core issues in the application of nano-carbon materials in lithium-ion batteries.
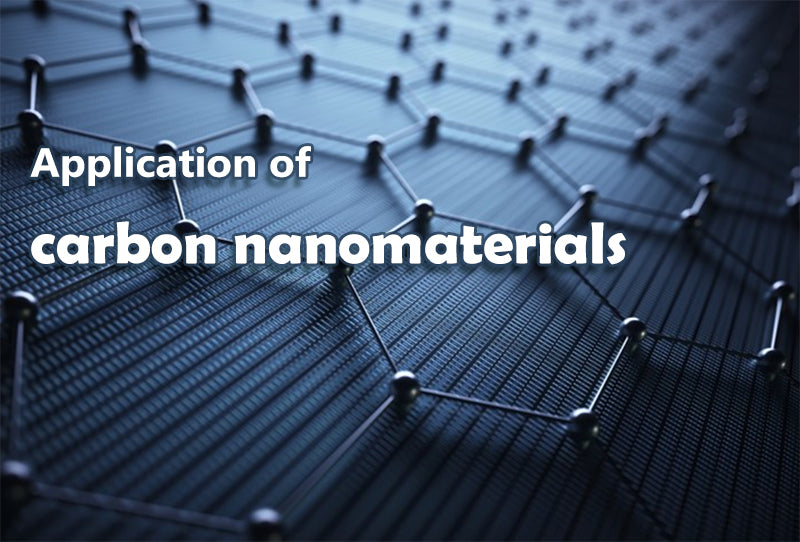
According to the migration path of electrons, the construction of a conductive network includes three approaches: carbon-coated current collectors, conductive additives, and carbon-coated/loaded active materials. Carbon-coated current collectors, coating or directly growing carbon layers on the surface of metal current collectors, using the conductivity and chemical inertness of carbon materials to improve the electrical contact between electrodes and current collectors, reduce interface contact resistance, and at the same time inhibit electrolyte corrosion of current collectors.
Conductive additives can build a continuous conductive network between active material particles and improve the electronic conductivity inside the electrode material. Nano-carbon materials are filled inside the electrode as conductive particles, which can build a uniform and stable electron transport network between active materials.
For active materials with poor electronic conductivity, the electron migration ability of the surface can be improved by carbon coating or carbon material surface loading, and the reactive active sites can be increased. The above three approaches have a positive effect on reducing the internal resistance of the battery, and any short board will lead to polarization.
Stability of SEI film
The electrolyte is thermodynamically unstable on the surface of the electrode, and a reduction reaction can occur and a thin passivation film is deposited, which is called a solid electrolyte film, also called an SEI film. The SEI film is a good conductor of Li+ and an electronic insulator, which can effectively inhibit the further decomposition of the electrolyte, so a stable SEI film is crucial to the stability of battery cycle.
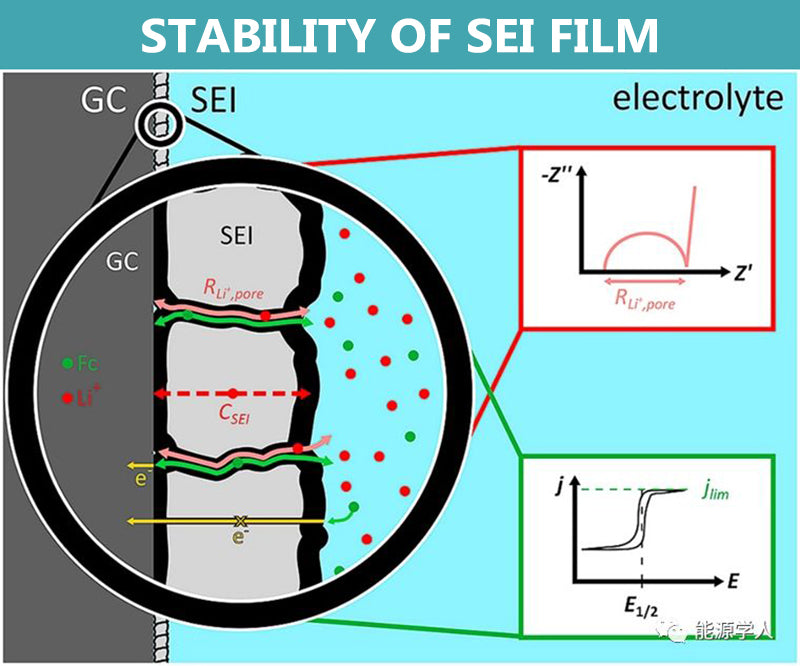
In the field of lithium batteries, silicon-based negative electrodes are one of the research hotspots. Silicon-based anodes have a high theoretical capacity, but there is a problem of volume expansion in practical applications. The shear stress and compressive stress generated by the volume deformation can easily lead to the rupture of the SEI film, exposing a new reactive surface.
The repeated rupture and formation of the SEI film not only consumes a large amount of active Li+ in the electrolyte, but also thickens continuously, hindering the diffusion of Li+, increasing the polarization, decreasing the first effect, and fading the capacity. The volumetric deformation can also cause part of the conductive additive to fall off, and the electrode and composite current collector to peel off, thereby destroying the conductive network.
Nano-carbon materials have the advantages of high specific surface area, surface functionalization, and controllable morphology. Taking advantage of its rich surface and structural properties, carbon coating of silicon is an important research idea to improve the stability of silicon anode SEI films.
Dispersion issues
Nano-carbon materials are conducive to building an excellent conductive network inside the electrode and improving the cycle stability of the battery. But there are problems of easy reunion and difficult dispersion. Nanomaterials have a high proportion of surface atoms and high surface energy, and usually exist stably in the form of aggregates.
Especially graphene, carbon nanotubes and other nanocarbon materials assembled with sp2 hybridized carbon atoms have a large number of π delocalized electrons on the surface, strong intermolecular forces, and easy stacking and winding, which greatly limits their excellent performance. Therefore, it is usually necessary to perform surface functionalization and dispersion treatment on nanocarbon materials such as hyperbranched carbon black, graphene, and carbon nanotubes to obtain a uniform and stable dispersion form.
However, this process consumes a large amount of solvent, and introduces non-conductive substances such as dispersants and thickeners, which brings problems in storage and transportation and battery safety. How to obtain a high-quality and uniform carbon network structure is a common problem faced by nano-carbon materials in the application of lithium-ion batteries.
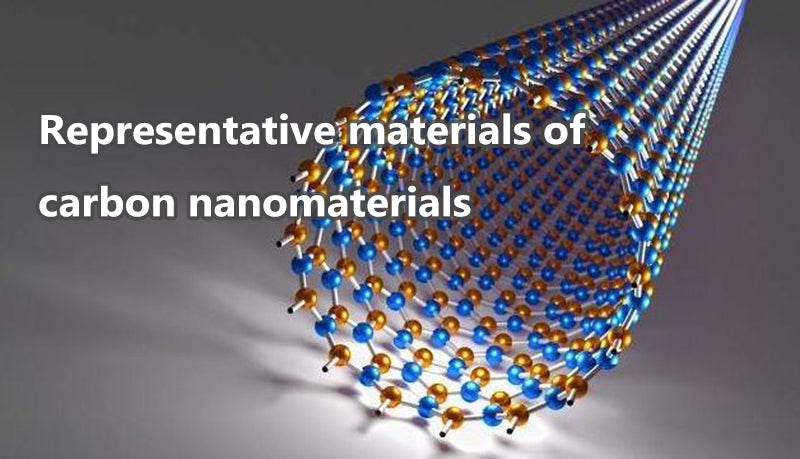
Representative materials of carbon nanomaterials
Fullerene
Fullerene consists of 60 carbon atoms through 12 five-membered rings and 20 six-membered rings, which is considered to be the most representative zero-dimensional nanocarbon material. In lithium-ion batteries, since fullerene is an allotrope of carbon, most studies regard it as the negative electrode material of lithium-ion batteries.
However, due to the single crystal nature of fullerene, the actual charge capacity is only 90mAh/g, and the reversibility of lithium storage is poor, so pure fullerene is not an ideal electrode lithium storage material. In order to obtain high-performance fullerene-based electrode materials, most studies have carried out further modifications and modifications on C60, such as doping, hybridization, and derivatization.
In addition to being used as electrode materials in lithium-ion batteries, fullerenes can also be prepared as coatings to improve electrode performance and as electrolyte additives.
Carbon nanotubes
Carbon nanotubes appear to be rolled up into tubular structures from graphite layers. According to the number of graphite layers, it can be divided into single-walled and multi-walled carbon nanotubes. Carbon nanotubes have excellent electrical conductivity and structural buildability, and have great application prospects in energy storage, catalysis and other fields. As an anode material for lithium-ion batteries, the capacity of carbon nanotubes largely depends on their structure and morphology (in the range of 300-1500mAh/g).
The difference in capacity among different CNTs can be attributed to structural factors such as chirality, diameter, length, defects, etc. Through post-processing such as ball milling, acid oxidation and metal oxide cutting, the reversible capacity of the electrode material can reach up to 1116mAh/g. However, it is still challenging to achieve high Coulombic efficiency with single CNTs as electrode materials due to the large structural defects and high voltage hysteresis of CNTs.
In order to obtain better electrochemical and physical properties, carbon nanotubes can be combined with active materials to form a composite structure. In composite materials, carbon nanotubes can form a stable interpenetrating conductive network, thereby shortening the lithium diffusion distance and realizing rapid charge transport.
Graphene
Graphene has many similarities with carbon nanotubes in structure and performance, including high specific surface area, abundant electronic states and good mechanical properties, and can be used instead of carbon nanotubes in many fields. In the field of energy storage applications, atomically thick graphene sheets with two-dimensional planar geometry are more conducive to electron transport than carbon nanotubes and can be more effective electrode materials.
However, graphene has problems such as low coulombic efficiency, voltage hysteresis, and poor rate performance, making it difficult to directly use it as an electrode material. Similar to carbon nanotubes, graphene can also be combined with active materials to form hybrid structures to prepare electrode materials with excellent performance. In addition to being used as an electrode material, graphene can also be used as a conductive coating and interface barrier to enhance the anti-corrosion and electrochemical performance of metal current collectors, and can also be directly made into current collectors.
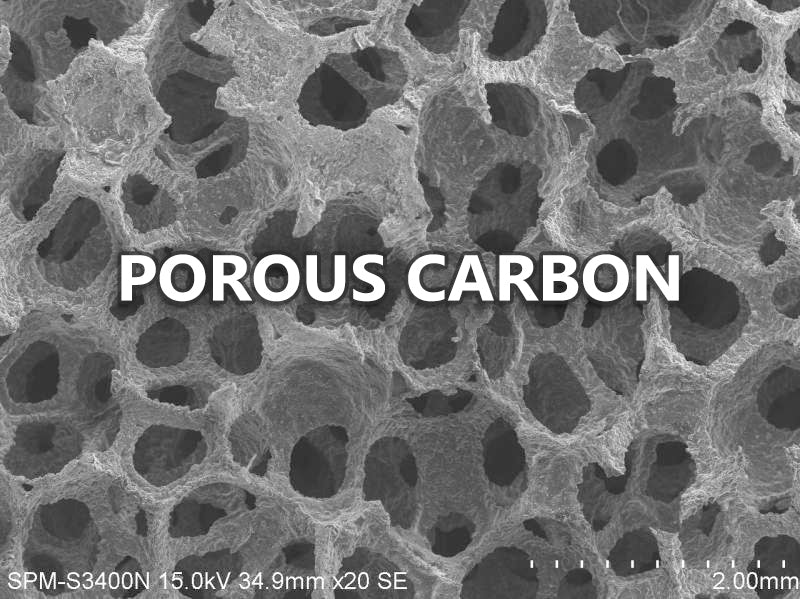
Porous carbon
Porous carbon materials are widely used in energy storage and catalysis because of their high specific surface area, controllable microscopic morphology, rich pore structure, good electrical conductivity, good stability and low synthesis cost. field. When used as the negative electrode of lithium-ion batteries, the high specific surface area of porous carbon enables it to combine more lithium ions and provide high capacity for lithium-ion batteries.
The multi-dimensional and complex pore structure provides effective diffusion channels for lithium ions and shorter The diffusion distance of lithium ions; defects such as vacancies and heteroatom doping can be used as lithium storage sites. The mechanical stress of volume expansion/shrinkage during lithium deintercalation is small, and the cycle stability is good. Therefore, porous carbons often exhibit better electrochemical performance than conventional graphitic carbons.
Porous carbon can be divided into three types according to the pore size, microporous carbon, mesoporous carbon and macroporous carbon. However, porous carbon materials with single pores have more or less defects. In order to improve the performance of porous carbons, hierarchical porous carbon materials with pore structures of different sizes, interconnected pore structures and combined in a hierarchical form have attracted attention. Micropores provide materials with a high specific surface area to enhance charge storage capacity, thereby increasing the capacity of lithium-ion batteries.
Mesopores provide fast channels for the transport of electrolyte ions, improving electrolyte penetration, and macropores provide shorter electrolyte ions. The diffusion distance promotes the diffusion of ions, and the capacity retention rate of high current is high. Compared with carbon nanotubes and graphene materials, which are widely used in self-supporting electrodes and current collectors, there are relatively few researches on the application of porous carbon materials in this area.
Summary
The development of nanocarbon materials provides an opportunity to design new energy storage materials suitable for lithium-ion batteries. As new carbon materials, they have many unique properties, including unique morphology, high specific surface area, low diffusion distance, high electrical conductivity and ion conductivity, controllable synthesis and doping, etc.
Therefore, nanocarbon materials have great application prospects in lithium-ion batteries with high reversible capacity, high power density, long cycle stability and high safety. However, carbon nanomaterials generally have disadvantages such as low initial Coulombic efficiency and voltage hysteresis. As a new type of material, it still faces some problems and challenges in practical applications, and there is still a long way to go before the real large-scale application.
Related article: carbon zinc battery vs alkaline, battery-grade lithium carbonate companies, lithium carbonate